Kyoku Gen Plasma(KGP)(Extreme Plasma Laboratory)
Summary
KGP group is working on extreme plasma phenomena with intense lasers to study the mysteries of energy production and transport in the universe. These studies have also opened up new frontiers in nuclear fusion science, radiotherapy and security diagnostics. Our research activity covers a wide range of theoretical and experimental studies on extreme plasmas, with particular interest in the efficient acceleration mechanisms enabled by ultra-thin graphene and nano-structured targets. Our work also includes engineering developments in target fabrication and high-energy particle diagnostics. These studies are performed under strong international collaborations between USA, UK, France, Romania, Taiwan, etc.
Research topics
1. Laboratory astrophysics
By recreating the properties of celestial objects under scaled conditions in the laboratory, we are studying extreme conditions such as the hot dense plasmas found in stars, strong electromagnetic fields that accelerate particle to the speed of light. High-power lasers provide experimental data that yields important information on astrophysics that cannot be obtained by satellite or telescope observation. Our recent experimental studies at ILE have revealed some parts of physical process in magnetic reconnection and related particle acceleration found as solar flares [1]. This is one of the successful cases of laboratory astrophysics. Many other attempts have been made to understand the physics such as collisionless shock and hydrodynamic instabilities, that could be the principle of high-energy particle acceleration in space.
[1] Y. Kuramitsu et al. Nature Communications, vol. 9. 5109 (2018)
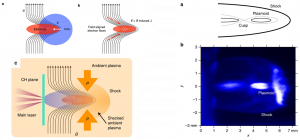
Fig. 1 (Left) Conceptual diagram of the influence of the ambient magnetic field and current generation due to the expansion of the laser-generated plasma. (Right) Observation of plasmoid generation due to magnetic reconnection [1].
2.Inertial confinement fusion (ICF)
One of ILE’s key goals is to demonstrate a self-sustaining thermonuclear fusion reaction, mimicking the process of energy production in the sun and stars. We have been working on an innovative approach to inertial confinement fusion (ICF), called “fast ignition”. Fast ignition scheme has the potential for higher energy gain at lower overall driver energy and cost. The key process is the localized energy deposition of energetic particles (electrons, protons, deuterons etc.) into a pre-compressed fuel. Our mission is to understand the fundamental physics of laser interaction with high energy density (HED) plasma, hydrodynamic instability, and relativistic electron generation and their transport inside dense plasma [2]. Our experimental attempts and modeling on these physics using a large-scale computer simulation have been contributing to important advances toward achieving “fusion ignition” in the laboratory by the fast ignition scheme.
[2] T. Gong, H. Habara et al., Nature Communications, vol. 10, 5614 (2019)
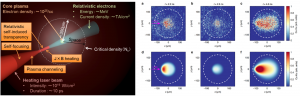
Fig. 2 (Left) Conceptual diagram of the fast ignition scheme. (Right) Observation of an imploded fuel plasma heated by relativistic electron beams [2].
3.Particle beam application
The advent of ultra-intense short-pulsed lasers has created new opportunities to produce energetic particle beams (electrons, ions, neutrons, x-rays) with favorable setup for future applications including radiotherapy, material screening, and nuclear transmutation. Particle acceleration by laser offers benefits in terms of cost-effectiveness and compactness in radiation confinement, providing much more compact design for particle source than conventional accelerators. With the technological advances in laser-driven particle acceleration, ion energy is now approaching GeV/nucleon, which is currently only available at large synchrotron facilities. The improvements in ion energy would also provide a bright source of neutrons by secondary nuclear reactions between ions and bulk nuclei. Our recent research on explosive detection by neutron radiography and activation analysis shows a bright prospect for laser-driven neutron sources, and motivate us to develop a compact neutron source [3], imaging device for neutron radiography, and high-resolution gamma-ray spectrometers for material screening.
[3] Y. Abe et al., Appl. Phys. Lett. 111, 233506 (2017)
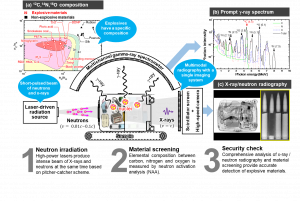
Fig. 3 Conceptual diagram of the security screening system based on laser-driven radiation sources.
Member
KURAMITSU Yasuhiro | Professor |
HABARA Hideaki | Associate Professor |
ABE Yuki | Assistant professor |